This is the last day of B.B.’s hunt, so he’ll start answering questions again on Thursday. Thanks for your patience.
by B.B. Pelletier
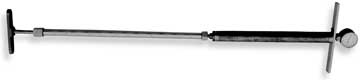
The test fixture Quackenbush made to ascertain pump efficiency. The pump attached in this photo is the smaller one with the 5/8″ piston head.
Empirical testing
I asked Dennis Quackenbush to tell me how much pressure a common hand pump can generate. He’s been making replica pumps for vintage big bore guns for several years. Dennis’ pumps are true to the old designs, except that they use synthetic pump seals. The old pumps had either a simple iron or steel piston that was lapped into the bore of the pump tube, or they used stacked leather washers on the end of the piston rod instead of a metal piston. The stacked washers were compressed by means of a nut so the fit could be controlled.
I’ve encountered several dozen antique pumps, and all of them were the type having the simple lapped iron piston. I only know about the stacked washer type from reading Air Guns by Eldon Wolff. He admits that the leather washer type is much rarer than the plain piston type.
Before Dennis conducted the tests, it seemed to both of us that neither vintage pump design could be the equal of one with a modern synthetic seal; so whatever pressure he could generate with a modern replica would represent a maximum for any vintage pump of the same physical specifications. That turned out to be an incorrect assumption, as we shall see.
We both agreed that the practical maximum force that could be applied would be the weight of the person doing the pumping. These early pumps had no mechanical advantage beyond that which is inherent in a single-stage mechanism. Although it would be possible to generate more force than one’s weight by pulling the base of the pump toward oneself or by jumping on the pump handle, it isn’t practical to do so–and it would be very hard to do it on a continuing basis.
Mechanical advantage is possible, and there are some vintage pumps that use it, but they’re rare compared to the bulk of the pumps we know about. The single-stage manual pump is the most common design encountered in vintage airgun equipment.
Dennis saw where I wanted to go with this experiment, and he took up the challenge enthusiastically. He used two different vintage-type pumps of his manufacture, plus the modern Axsor pump to check efficiency. He provided the following data.
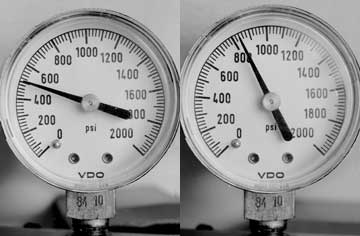
I pumped Quackenbush’s test fixture to 500 psi and left it there for four months without air loss. Then, it was pumped up to 840 psi. Because I weigh more than Quackenbush, the higher pressure was easy to obtain.
Test of air pump efficiency
Quackenbush connected the pumps to a nine-cubic-inch test reservoir, to which he had attached a pressure gauge. He then pumped up the reservoir with each pump and counted the strokes required to get to certain pressure levels. He repeated this experiment three times to verify his figures, which is in the box below. Naturally, when you consider the number of pump strokes required to build pressure in the 5/8″ diameter piston pump, he’s not going to repeat each test 30 times! But, in the repetitions he did, the results were close enough to make the figures believable. If anyone doubts the data, they can buy a pump from Dennis and repeat the experiment themselves.
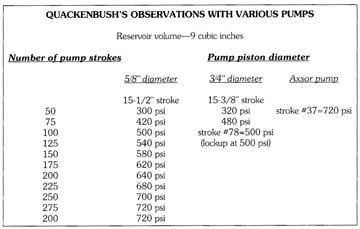
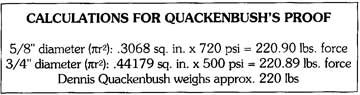
Next, he made another 5/8″ diameter pump without a seal to test the effectiveness of something truly vintage. I felt sure he would not be able to reach the same pressure as a pump with a synthetic seal. This one had only a tightly fitted piston with a thin film of oil to seal it. The results were quite surprising! The pump with no seal went all the way up to the same pressure as the pump with the synthetic seal. Proving, once again, that we aren’t as smart as we think.
Actually, it’s incorrect for me to say this pump has no seal, for the oil film seals quite well. Dennis achieved 720 psi with the plain piston pump, using approximately the same number of strokes as the other 5/8″ diameter pump that had the seal.
Then, on a suggestion from one of his airgunning friends, he tried “rapping” the air into the reservoir–similar to the method used with the Korean-built Yewah Triple B Dynamite shotgun. Rapping means imparting extra momentum to the pump through inertia. With this method, Dennis raised the pressure ceiling of the 5/8″ plain piston pump to about 810 psi, but at a high cost to his personal well-being. He said his wrists hurt so much from doing the rapping just once that he had to recuperate for several days thereafter. So, rapping, while possible, is not a practical way to fill vintage reservoirs to higher pressures, and it’s doubtful that anyone ever did it more than once.
One additional thing he did benefits this study even more than these test results. He noticed that the piston diameter relates directly to the total pressure achieved. By itself, this isn’t such a great discovery because Cardew already published similar data in The Airgun from Trigger to Target. What Quackenbush did for us was remove the complex mathematical formulae from Cardew’s work and substitute simple equations in their place.
Cardew says that 1,000 psi might be achieved by a heavy man. Quackenbush demonstrates that the pump stops at a point very close to the weight of the person doing the work…when the formula is applied.
Quackenbush’s proof
What the data show is that it’s possible to calculate the maximum pressure for any single-stage air pump by simply dividing the weight of the pump operator by the area of the piston head. There should be some loss of efficiency due to friction in the pump, but this is most probably offset by some imprecision in determining the person’s body weight:
This allows us to build a “theoretical pump” that can generate whatever pressure we desire within the limits of physical laws. For example:
250 lbs. divided by .19635 = 1,273.24 psi
150-lb. operator using a pump with a 1/2″ piston
150 lbs. divided by .19635 = 763.94 psi
Notice that the stroke of the piston does not enter into this calculation. That’s because the length of the stroke determines only how much air is being compressed. It doesn’t affect the highest pressure that can be achieved. A longer stroke will compress a greater volume of air; a small-diameter piston will allow it to build to higher pressure.
Up to this point, the term single-stage has been used when referring to the pumps of old. Single-stage simply means that the pump is compressing air in one direction rather than on both strokes. In all cases, the direction for compression of a single-stage pump is the down stroke. The upward stroke sucks in more air for the next downward compression stroke.
The modern high-pressure air pump from Sweden, in contrast, compresses air in both directions. It’s really two pumps nestled one inside the other, both housed inside an outer tube. [No, it’s actually THREE pumps, nestled inside one another. And there is a new Swedish pump that claims to be four pumps!] Both pistons have a small piston diameter. The center of the larger pump is occupied by the smaller pump, so the AREA of the two piston heads is comparably small. The larger of the two is the second stage, which operates on the downward stroke–the direction in which the greatest force can be applied. The upward stroke sucks the air into the pump and pressurizes it slightly to begin with, thus making it ready for the second stage to compress it to a very high pressure level. This pump pressurizes a greater volume of air to a higher pressure than its small piston would normally allow because it acts as though it’s twice as long as it is.
If a single-stage pump were made with a very small piston–perhaps 3/8″, what sort of pressure might be possible from a 150-lb. operator?
Of course, this small piston pump would take a lot longer to fill a reservoir, so we might increase its stroke to help matters. That gives us a long, thin pump that would eventually become too long to carry conveniently. Also, the thinner the pump piston, the thinner the piston rod; although the piston HEAD might be able to compress very high pressures, the rod that connects it to a source of force will eventually become too thin to bear up under the strain.
From this discussion, we see that Cardew was correct. It’s possible to generate 1,000 psi and even more with a simple single-stage air pump.
Not only do vintage pumps begin to max out at pressures much lower than modern pumps can achieve, the guns being pressurized cannot deal with air pressures anywhere near these high levels.
The large valve contact surfaces of vintage big bores constrain them to use lower-pressure air. With the state-of-the-art technology available to 17th century airgun makers, it was all they could do to seal valves against pressures ranging from 400 psi up to 650 psi. They used horn and leather to accomplish as much as they did. Today’s use of hard synthetic valve heads bearing on thin areas of contact on precisely machined steel seats was simply beyond anything they could achieve.
Also, they lacked the homogenous materials from which to make reservoirs to contain the higher air pressures. Even if they’d been able to make the valves and pumps to work at higher pressures, the reservoirs would have held them back.
Again, I turn to the documented studies that have been done to support this statement. This time, there’s even less information than before. The number of ancient airguns that have been intentionally tested to destruction is almost zero. A fair number of them have blown up from too much pressure, but that was accidental; and apart from a big boom and possible injury to the operator, there isn’t much to go on. Cardew does mention one of the folded and brazed butt reservoirs that was tested to destruction.
Amazingly, the antique folded-iron reservoir held until 6,000 psi was developed. When it finally did blow, only one rivet popped, resulting in a controlled exhaust rather than a catastrophic explosion.
When such tests are conducted, the vessel being tested is never filled with air for fear of an explosion. Instead, oil or water is forced in under pressure, so the failure can only result in a safe leak. Unfortunately, this test is exactly the kind of report that a careless person will cite when trying to operate vintage equipment in an unsafe manner.
A case in point
Modern aluminum paintball tanks are designed to contain CO2 safely. CO2 has a pressure determined by temperature. At 70-degrees F, it’s just under 900 psi. When the temperature rises to 95 degrees, the pressure increases to around 1,100 psi. Because of this variability, and because of the possibility of even higher temperatures, such as when a tank is stored in a car on a warm day (130 degrees is easily possible), the manufacturers rate the tank up to 1,800 psi. That’s the number they put on the label on the tank for everyone to see.
Now, along comes Mr. Airgunner, and he reads this number. Okay, he thinks to himself, if this tank is rated to 1,800 psi, it’s safe to pressurize it to that level. He fills it with air instead of CO2. The air tanks rated this high cost much more than the CO2 tanks (wonder why?), so using a paintball tank represents a real savings. If he does this, the tank is filled right up to its design maximu–a level that was engineered by the manufacturer to handle an emergency only. Because CO2 pressure varies with temperature, they make the tank to withstand a higher pressure in case the temperature ever rises unexpectedly. It isn’t expected to withstand that pressure all the time, even though it’s engineered to do so. It’s expected to hold a pressure of around 900 psi when confined. Some folks go by the numbers without appreciating that they’re actually subjecting these bottles to a 100 percent overfill. It doesn’t end there.
If one person is willing to do that, what’s to stop another from pressurizing the same tank right on up to 3,000 psi with the same fill equipment? Maybe he’s been using paintball tanks this way for a long time, and there’s never been any trouble before. “After all,” he says, “these things are over-engineered, anyway.”
Yes, they are. And, at 3,000 psi, you’re more than 2/3 of the way into that safety margin. Standard pressure vessels are engineered to not fail with less than four times their standard working pressure–which you’ll remember is 900 psi, nominally. Blowup can occur any time after 3,600 psi for a tank having a 900 psi standard working pressure. That’s for a new tank. Who’s to say at what point an old abused tank will let go?
But tanks have safety valves (burst disks), don’t they? Again, yes, they do. Do you want to trust your life to a small piece of metal that’s been sitting in the tank for years? What if the kid at the plant decided to use heavier sheet metal when your tank was made? What if there was a goof when the company placed the order and a stronger material was used without anyone’s knowledge? What if someone in the field “fixed” it before you got it (perhaps to keep it from rupturing so easily)?
If modern paintball tanks represent a danger from over pressurization, what about vintage air reservoirs? They don’t have burst disks or any other safety devices, plus they’ve been lying around for decades and centuries with their dubious metal walls containing who knows what kinds of pressure.
Also, the old reservoirs intentionally had grease smeared on the walls near the valve to catch and retain dirt and dust. At 500 psi, nothing is going to happen; but when you get one of these oldies up to 2,000 psi and more, that grease is going to vaporize into a highly explosive gas. Just ask a dive shop what happens when petroleum-based lubricants are used in scuba tanks. Boom!
When we work with precharged airguns of any type, we want to obey all the safety rules that pertain to them. When the airguns are also large bore, we want to be especially careful because they’re so powerful.
Part 3 will demonstrate how pressure relates to velocity.
Just to let some of you know, I have a fairly accurate temp. gauage that I bought from a snap-on dealer used to measure A/C temp from the cars vents. It goes from something like 0-200 degrees. Where I live, it’s nothing for it to be 95-100 in the summer. After sitting in my truck in the sun all day that temp sensor typically reads 155 degrees. From SavageSam
I’ve actually used the sun to “recharge” my 3,500 # tank… when it got down to about 1,800, I put in the sun where I was shooting and I got a few xtra fills for the Discovery, I guess it gained about 20% on the 80 degree day, in the sun in about one hour..
Don’t try this with an old tank, or one that is near full.. just an emergency measure, in the field maybe.. Or better still, don’t do it at all… just call it a studident about to happen…that didn’t…yet..
Wayne
Ashland Air Rifle Range
Volvo & Vince
Thanks for the advice on using the Yellow classified, boy did I get action on those used guns..
Since we are talking about PCPs, and ones that do special things…(or at least I am, I always do) I might have found another Air Arms S410 that gets a lot of shots like my .177 sidelever, which gets 130 shots over 760fps with 8.4 JSB on a fill of 205 bar..
I traded with a new found friend in FL., Dan…. I'm sending off my TX200 carbine and HW-77 to him for a like new, but not side lever, (rear bolt action) Air Arms S410 in .22 cal… It's like a big brother for my .177
Dan got it from someone else, and doesn't like being tied to the scuba tank, when his wife can just walk off into the woods with the TX200 carbine they have already.. So now they can be "twinnezzs"..
How lucky Dan is, to have his wife shooting with him..
Anyway, if you want, look at the string of shots this guy posted on what that .22 cal Air Arms S410 did, and how handsome he is.. a perfect match to my Walnut .177.. I'm overjoyed!!
We should both have our new treasures by the first of the week..maybe Saturday if the PO does well..
Billy sent off the USFT too, so what a weekend I should have opening boxes and testing the air rifle Tim and LD did their best to get ready to win the world title in 2005, Billy couldn't make it that year because of medical issues.. it took 12th in the wind last year in the Ireland world championship contest, in the hands of Paul Cray.. Maybe someday it will get that title back for the United States.. I'd love to see Billy Lo win it for us.. with MY gun!!!
here is the link to the S410 .22 cal
http://www.network54.com/Forum/79574/message/1221266159/Air+Arms+AAs410+10-shot+.22+with+incredible+walnut+stock.+41+FPE+with+Eun+Jins!
Wayne,
Ashland Air Rifle Range
Good morning B.B. How’s the hunt coming? Are we perchance hunting some of the time with a vintage air gun being pumped up by a retired sumo wrestler:)? Did anyone try using a piston with rings? How about some info on the valve in the tank and or the gun. Thanks Mr B.
B.B.,
Great blog! I had no idea a steel-on-steel piston could achieve those kinds of pressures.
I am curious about what kind of check valves were used in these pumps.
Thanks,
Lloyd
Of course the other thing you have to keep in mind is that in the 17th or 18th century people were generally smaller than they are today. Royalty might have someone to pump their gun up but a soldier or explorer would have to do it themselves and from watching the History channel a 150 lb man would have been larger than average which would seem to indicate that an operating pressure of 500 psi or so would be a reasonable max.
Sam
Perhaps two people pumped at the same time to get more pressure.
BB,
I’m lovin’ this series! Looking forward to the next installment.
.22 multi-shot
Why wouldn’t they have the horses sit on the pump and get up and sit and get up and sit…. 🙂
It seems like they could’ve made a methane collector with diaper as well.. the first semi-auto MO2…
Wayne
BB,
I can see the limitations for portable pumps, but it seems like more pressure could be developed by using a lever, e.g. with the pump rod between a fulcrum and a handle. These kinds of setups were common on water pumps, I believe.
My thoughts on pumps,
Well they make my feet hurt and crowd my toes. Oppie, wrong pumps.
The 4 stage Swedish pump does make pumping to 3000 psi no more difficult than the 2000 psi with the Air Force Discovery pump. I did not try any serious testing as I assume most folks probably graduate to using scuba tanks – and each rifle needs its own connecting pieces – which I was too lazy to change.
The FX pump did include two moisture filters, the Discovery none. Not sure if they are needed or not?
Maybe if it is a long Winter I will try and compare the efficiency of the two pumps by filling the same rifle with them.
Wayne, that is one nice piece of wood. I’ll be in the corner with my Discovery wiping my eyes. : )
Volvo
Wayne,
It’s perfectly okay to put your tanks in the car’s heat, as that is not going to multiply the pressure to potentially hazardous levels (the kinds of multiples that BB is writing about). These tanks, even when years old, will easily handle the increase of pressure that 135 degrees and higher will bring.
I always keep my CO2 tanks {from 12.5 gram to 20 ounce) in the furnace/hot water heater room year round, where it often is warm and sometimes up to 95 degrees (I live in Northeast), and by also adding a battery operated heated sock on the big CO2 tank to maintain its warmth to some extent just before going out, I can shoot without problem in the cold weather.
I’d be surprised if anything ever blows up because I see how old the tanks are and whenever I get them refilled the refill guy has to follow the legal statutes which require periodic testing followed by destruction of the too-old tank.
I really think that what BB says about not trusting your face being blown off because some guy in a factory making some metal part or the previous guy who owned the gun did not do the correct procedure has a lot of validity when pushing the envelope of a manufactured part.
On the other hand, think about all the old, worn, metal parts/brittle rubber hoses/corroded and rusted bolts in so many of the 10+ year-old cars/light trucks on the highways and streets, as they whizz by several feet from your own car (or body, if you are a pedestrian) every week at 70 mph. Now, your life is dependent on all those parts continuing to work, and it seems that they all do, as I rarely hear of a car spontaneously destructing, even though I imagine that the engineers left detailed instructions on maintaining and inspecting the cars after they left the factory, instructions that a significant portion of the population fail to adhere to as they push their 13-year-old clunkers another year, praying that they pass the next inspection (which from an engineering point of view is a joke).
So don’t worry. Go ahead and push those modern CO2 tanks into the heat and enjoy a few more shots.
– Dr. G.
Volvo,
Yes, that is a handsome dude, in the English walnut suit…. very nice grain.. It looks like it might be from the same tree as my Air Arms S410 .177 side lever.. :)I know they were meant to lay side by side in my inventory… and if the specs. on shots per tank are for real.. I've got an other special gun… I think some of these have a different valve… but "I know nothing" as Schulz says..
Dr. G.
It seemed pretty safe, to set a tank approved to 3,500.. (and so safe to 4,500 I guess) in the sun when it gets to 1,800… and get a few more shots per tank… these are air scuba tanks… so even more safe to play with…
thanks for your ok to continue… I'll let you know if I get my face blown off… 🙂
Wayne
AARR&R
Wayne, God forbid, but if it should happen, dibs on the S410. Mr B.
mr b.
ok, your on the list of..
a long, long, long, long, loong. list
but your on the top…
Wayne
Tanks-
In case you are curious——
Scuba tanks are hydro tested at 167% of rated pressure, so a 3000 psi tank is tested to 5000 psi. In a nutshell, the tank is filled with water and the volume is noted; pressure is then brought up to 167% and the new volume is noted. Then the pressure is dropped back to zero and the volume is checked to see if the tank returned to its original volume. If it did, then everything is cool. If the tank has taken a permanent expansion of greater than a few percent, that means the metal has exceeded its yield point and that is bad news.
Be careful and don’t overfill anything, But do remember that the tank was tested at a much higher pressure.
Lloyd
Volvo,
I would be interested in those test results. I’ve especially been wondering about whether a moisture trap does any good.
Wayne,
I tend to play things safe, but I wouldn’t think it would be a problem letting heat bring the tank up to its working pressure. Just my 2 cents.
.22 multi-shot
.22 multi-shot,
I think the moisture filters are just to keep condensation out of the rifle and prevent rust. The discovery pump is supposed to have a filter, which I can only assume is internal.
The 4-stage FX pump has two external filters that are supposed to help remove moisture.
Since it would rust from the inside out, like my 1970’s era Camaro did, it would go unnoticed until it was too late.
As far as the efficiency of the pumps, I’m curious also. I am wondering if it would be fair to compare how many pumps it takes to add 1000 psi in two different rifles?
Bg – farmer or anyone else more technically inclined – any input?
Volvo
Wayne, I envy your weekend opening those boxes. Most nterested in hearing about your USFT rifle. Mr B.
Volvo,
I agree with you about the point of the moisture filters. I’m assuming PCP’s don’t have a drain valve like many air tanks (e.g. on a compressor) do. If not, it would be especially important to keep water out of them.
My curiosity about moisture traps isn’t about whether it is good to keep water of the gun. I would agree it is good. I’m wondering whether they really work or not (and to what extent)?
Someone I talked to about pneumatic tools told me that people tend to put the moisture trap in the wrong place. If the air is heated, the moisture is evaporated and is difficult to trap. I would assume that the hand pumps have the trap on intake before the air is heated by compression.
.22 multi-shot
You fellows are worrying just too much about these things…moisture, heating tanks, making too much pressure…it’s a good thing that the manufacturers have almost perfected these air guns, or god only knows what you would be worrying about next!
What, Me Worry?
– Dr. G.
Here are a few reasons I am concerned. This does not mean that we should stop using pressure vessels, but that we need to be informed about proper usage (which BB is doing).
http://biobug.org/scuba/scubatank/
http://www.sealancers.org/Articles/tanks/cscuba.htm
http://www.cdnn.info/safety/s040729/s040729.html
http://www.projo.com/ri/tiverton/content/EB_TIVSCUBA14_06-14-07_VQ60O4B.2f0bc7c.html
That means only filling to working pressure (3500 in Wayne’s case), keeping moisture out of pressure vessels, not using petroleum based lubricants in PCPs, knowing about faulty alloys (Aluminum 6351-T6: avoid tanks made with it), etc.
I would like to “live long and prosper” with my wife and kids!
.22 multi-shot
.22 multi,
Several moths ago, a friend gave me an out-of-hydro aluminum tank. It failed eddy current and the shop gave me a discount on a high pressure steel tank to replace it. I thought it seemed like a scam. Reading your links, and checking the tank, I see that it was an 80’s vintage DOT AL3 tank. Yikes! Glad its out of service.
Thanks for the info.
Lloyd
Lloyd,
Glad you found the information useful. I sure wouldn’t want to be in an accident like that!
.22 multi-shot
I have an off topic question. I know that if you leave a spring powered air rifle cocked for a long period of time you can damage the springs. But the question is how long can you leave it cocked without causing any damage to the springs?
Dale,
I tested that when I wrote the R1 book. I tested four springs by leaving them cocked for a month and testing them at different times during the month.
Read this report (which was written before I revealed who I am):
/blog/2006/5/how-long-does-a-mainspring-last-part-2/
B.B.